Follow me on Twitter if you want to discuss this essay, my other essays, or anything else having to do with science and biotech. If you’d like to get notified when I publish new essays, sign up for my mailing list, which I literally only use to alert people when I publish new essays.
A lot of money and effort has recently been poured into aging therapeutics. Altos Labs, for instance, has raised $270 million dollars in the last year for pursuing “cellular longevity”, or making individual cells live longer (in the hopes that will make people live longer as well). Other aging startups are also exceedingly well-funded, like Loyal, which has raised $27 million to try to extend the lifespan of dogs before even making it to the clinic.
This is a lot of money, even for biotech. Most traditional biotech can make it to evidence of efficacy in humans for around $30 million total, and drug repurposing efforts (like my own) can do so for $5-10 million.
The sheer amount of money (coming largely from the tech crowd) being thrown at aging has, understandably, resulted in a lot of hype. I got my news of Loyal’s Series A from TechCrunch, for instance, covered in the same tone as any buzzy new app’s Series A. TechCrunch, for the record, didn’t even mention the name Moderna until Moderna was already in Phase 3 trials for its COVID vaccine.
I don’t think these anti-aging efforts are necessarily doomed to fail. I do think, however, that their public announcements and even their private assumptions massively underestimate the difficulty and complexity of reversing or stopping aging. Aging is biology, and biology, like reality, has a surprising amount of detail.
If I try to discuss aging or reversing aging in general, though, I’m liable to get stuck in generalities, which makes it hard to drill down into the sort of details that matter. So, instead, I’d like to discuss in detail a very specific part of aging: graying. Only then, after we discuss graying, can we start to get a sense of the difficulty and complexity of aging research.
Graying is a too often overlooked part of aging in general, in my opinion. While it’s easy to ignore it because it’s not part of the aging process that bothers us (George Clooney has basically his entire career to thank for it), it’s actually an incredibly useful part of aging to study.
First, almost every mammal grays as it ages and almost every mammal that is gray is old. That means graying is an incredibly conserved part of aging, as close to a visible biological clock as one can get.
Second, as I’ll discuss more below, not only do we know how to induce graying, we’ve actually accidentally reversed it as well. This gives us vital clues as to what can control the process of graying, clues that we don’t have for aging in general.
Third, graying is highly visible and indisputable. Advances in the aging field or aging-related diseases fields are frequently disputed because metrics aren’t universally agreed upon. It’s difficult to tell if a mouse has been cured of Alzheimer’s when mice don’t get Alzheimer’s to begin with. Scientists are forced to resort to saying, “We did something to this mouse that looked like giving it Alzheimer’s, and then we did something else that looked like it put the mouse back to square 1”. This is not the case for an anti-graying intervention.
Finally, interventions that affect graying can be done without harming the overall health of the individual, which is helpful both from an ethical standpoint and a logistical one (i.e. we don’t have to continually get new lab animals). One could even imagine some dedicated researcher experimenting on himself to reverse his own graying. If he messes up and does the opposite, it’s not that big of a deal.
I’m not the first person to notice these advantages, of course. A lot of people have thought this way, and there’s a robust literature of people experimenting with graying and anti-graying techniques on hair in culture, in animals, and even in humans. All it takes is for a scientist with a pair of tweezers to say to their graying colleague “come here for a second” and they can get another experiment up and running, which is a far cry from how complicated it is to run an Alzheimer’s experiment.
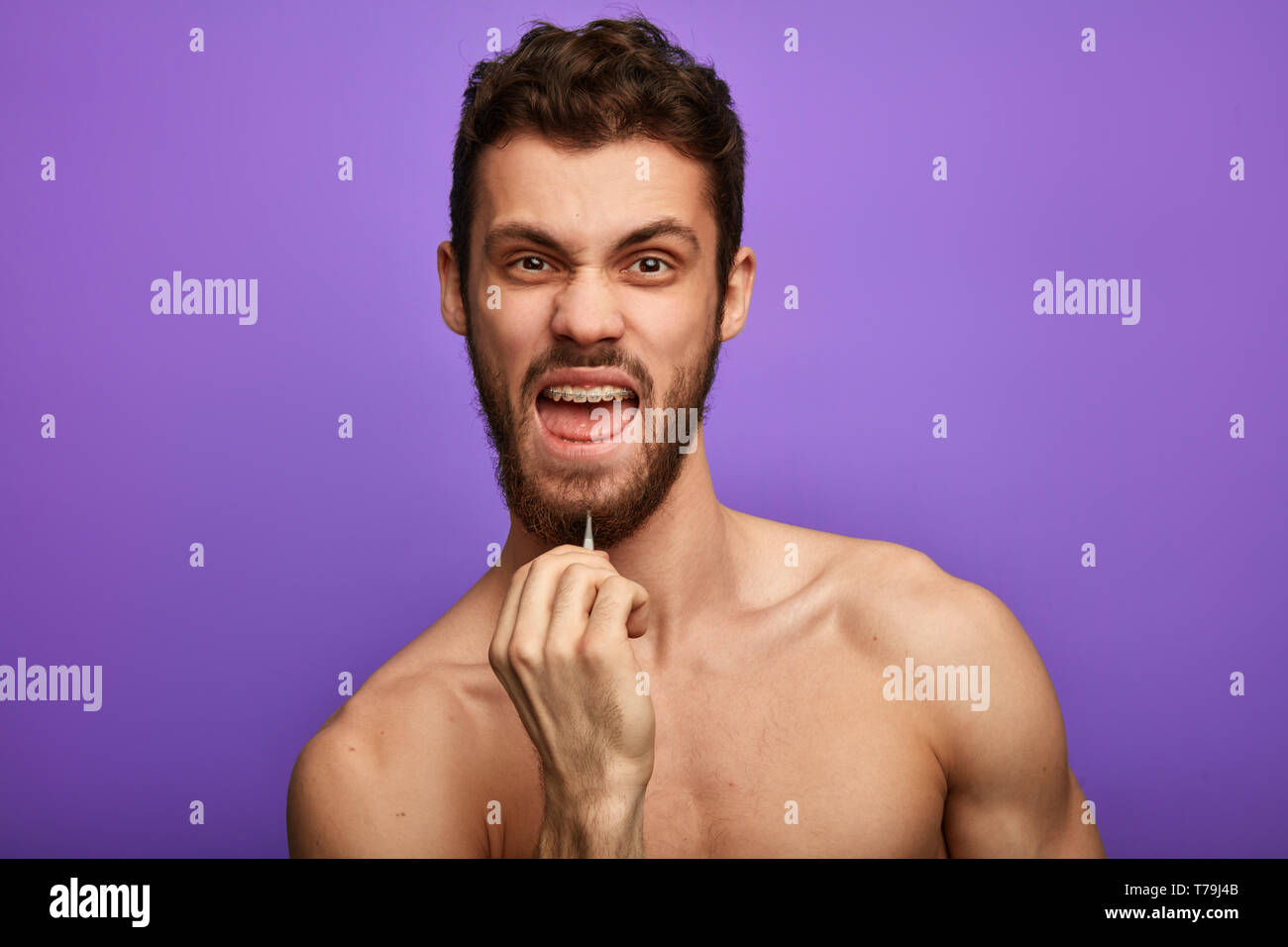
But that’s precisely my point. Despite these evident, huge advantages to studying graying, graying is still really difficult to understand and impossible to cure. For the rest of this essay, I’ll discuss what we know about graying, what we don’t know, and where the field is going from here. Then I’ll try to put it back in context with the rest of aging research, so we can get more context for how difficult aging research is.
Now, if you don’t feel like reading too much more, I’ll give you the summary. The two big things we know about graying are most of how it happens and how it’s been accidentally reversed. The biggest things we don’t know about graying are why it happens and how we can intentionally reverse it. If you’d like to know more detail, read on. Also, I should mention that I’ve relied a lot on this excellent review paper for explaining more about the biology of graying.
Anyway, here’s what we know about how it happens.
Here’s the normal cycle of a hair follicle. Over the period of several years, the hair grows out from a follicle, detaches, and then grows again.
Hair gets its darkness from melanin, like skin. This is produced by melanocytes that surround the bottom of the hair shaft, which is known as the matrix, as seen below. The process of producing melanin occurs only during anagen, as does the process of producing more melanocytes from the melanocyte stem cells that surround the middle of the hair shaft, which is known as the bulge. During anagen, melanocytes migrate from the bulge to the matrix so they can start producing melanin. After anagen, the mature melanocytes in the matrix apoptose, or die.
Melanocytes and the lack thereof are key to the graying process. Gray hair follicles have fewer melanocytes at the start of anagen (and those melanocytes may be worse at producing melanin), while white hair follicles have almost no melanocytes or melanocyte stem cells.
The essential cause of graying seems to be, then, that melanocytes for some reason stop coming from the bulge to the matrix, or at least stop surviving and proliferating in the matrix. This results in a lack of melanin making its way to the hair shaft. After this happens, this is weirdly followed by the melanocyte stem cells also dying. Why this sequence of events occurs and not the reverse is one of the big mysteries of graying.
Part of the reason why it’s difficult to understand this sequence of events is that the normal cycle of melanocyte stem cells -> melanocytes -> melanin is much more complicated in life than it seems on paper. For one thing, it’s controlled by many, many hormonal regulators. Here’s a short list: ACTH, adrenocorticotropic hormone; α-MSH, alpha melanocyte-stimulating hormone; BMAL1, brain and muscle ARNT-like 1; CRH, corticotropin-releasing hormone; c-KIT, tyrosine protein kinase Kit; c-Met, tyrosine protein kinase Met; FGF, fibroblast growth factor; GR, glucocorticoid receptor; HGF, hepatocyte growth factor; HPA, hypothalamus–pituitary–adrenal axis; MC1R, melanocortin receptor; MITF, microphthalmia-associated transcription factor; NGF, nerve growth factor; PER1, period circadian regulator 1; POMC, proopiomelanocortin; SCF, stem cell factor; T3, triiodothyronine; T4, tetraiodothyronine; TRH, thyrotropin-releasing hormone; TrkA, tyrosine receptor kinase A; and TSC, tuberous sclerosis complex.
Any one of these can affect the pigmentation process. For example, nerve growth factor (NGF) is, as you might expect, a growth factor. It promotes the growth and proliferation of melanocytes. It does so by agonizing TrkA. If you grow a hair follicle in vitro and add in NGF, you can make the hair shaft dark with melanin long after the control hair factor has gone gray.
Is that then a solution to hair graying? Well, no. First of all, completely removing a cell from all hormones is not what causes hair graying in people. NGF never goes away in people. If it did, we’d be pretty screwed, because it’s used everywhere in the body (e.g. to promote nerve growth). Second of all, and more importantly, hormones are never quite so simple as more=better. While in this experiment 5 ng of NGF kept the hair shaft dark, 50 ng of NGF actually made the hair follicle lose pigmentation faster than the control.
At this point, I hope you’re starting to get some sense of the complexity of all of this. People who claim that aging is “just” one thing or “just” another radically underestimate the complexity of how cycles are controlled in the body. These scientists above understand what NGF is for in terms of hair darkening, are able to grow unlimited hair follicles in culture, and can add as much or as little NGF as they want. They still don’t know how much NGF is appropriate for an individual hair follicle on the human head in order to keep a hair strand dark, or even if a lack or excess of NGF is a major cause of graying. This stuff’s really difficult!
The other major piece of knowledge that we have in our arsenal with regards to graying are reports of sudden reversals of graying. Unfortunately, despite their value, these cases are woefully underexplored. They mostly just exist in reports from random doctors. This is a pity, because there’s not really an equivalent for any other piece of aging: nobody randomly gets cured from Alzheimer’s or randomly gets their joint degeneration turned to normal. If there are any real-world consequences from this essay, I’d hope that an exploration of these cases is one of them, because they are fascinating.
Some of the most reliable reports of spontaneous hair repigmentation come from immunosuppressants, including secukinumab, an anti-interleukin-17 therapy; adalimumab, an anti TNF-alpha therapy; and corticosteroids. Interestingly, the darkened hair growth persisted for both the adalimumab and the corticosteroids at least 20 and 12 months after the cessation of therapy, respectively (there was no cessation of secukinumab). While this isn’t longer than the 3-5 years of the total life cycle of a hair follicle, it does suggest that there was some immune component to hair graying in the first place. Immune cells attacking and killing melanocytes might be a common cause of hair graying.
There are also other reliable reports from other cases, but they’re even harder to explain.
Two patients with porphyria cutanea tarda experienced hair repigmentation concurrent with their other symptoms. One patient had continued hair repigmentation 9 years after treatment of her porphyria cutanea tarda resolved, which means that the repigmentation lasted beyond a hair follicle cycle. Porphyria cutanea tarda is caused by a deficiency of an enzyme that participates in the creation of heme, and results in increased photosensitivity.
Porphyria cutanea tarda also results in (and is usually diagnosed by) an elevated level of ferritin, a protein that stores iron. One can maybe imagine how excess iron could result in hair darkening, but it’s hard to imagine how that could then persist after the ferritin levels returned to normal.
There was also a report of erlotinib, a tyrosine kinase inhibitor, spontaneously causing hair repigmentation, but only on the top of the head. Interestingly, this evidently occurred mid follicle cycle, as the root of the hair was dark, while the tip of the hair remained gray. Tyrosine is a promoter of melanogenesis, so some sort of connection is suggested there, but it’s difficult to know why that would only occur at the top of the head.
The reverse happened with acitretin, where the crown of the head was gray but the occipital region turned black. Acitretin is closely related to vitamin A, and used to treat psoriasis. Vitamin A deficiency and excess can cause hair loss, likely by interacting with the hair follicle stem cells and sebaceous glands. The effect on hair stem cells is suggestive, but, again, it’s really difficult to explain why this would only affect the occipital region.
Trying to summarize all of these cases as what can reverse hair graying is difficult. The most obvious answer is that hair graying must be caused by many factors, and what reverses one person’s hair graying might not reverse another. The challenge for researchers, then, would be to predict which people would be affected by which interventions, and then figure out which parts of the intervention are actually necessary.
And that brings us back to the biggest thing we don’t know: why any of this happens. We don’t know why melanocytes stop producing melanin or migrating down to the matrix. We don’t know why melanocytes die before melanocyte stem cells, or why this is so conserved across mammal species.
And, because we don’t know why, we still are not remotely close to someone with gray hair being able to walk into a doctor’s office and walk out with black hair. In fact, we’re still not remotely close to being able to consistently reverse graying even in a mouse.
Our difficulties with these presage a difficulty with reversing aging as a whole. Aging is made up of a bunch of different systems: aging of the skin, the heart, the brain, the immune system. All of these systems age and get worse at their jobs, which is why so many diseases have aging as a risk factor. We can safely assume, too, that all of these systems are at least as complex as a hair follicle, with incredibly long lists of hormones that interact with each other in confusing ways.
But, unlike with graying, aging scientists don’t have the luxury of just looking at each other’s heads, looking at case reports of sudden reversals, or even just being able to visually confirm whether a treatment worked. Testing whether an aging treatment worked in the heart or in the brain is a lot more complicated than that. So, there are a lot of hard slogs ahead, and I have no doubts that the same sorts of weird paradoxes that pop up in graying (e.g. melanocytes disappearing before stem cells) will pop up in general aging, too. They’ll just be harder to spot.
So, to those scientists with millions of dollars who plan to cure aging within 10 years, I wish you best of luck. But, I urge you: as you work in your labs, please take a second to stop and look at the grayheads around you. Ask them for their wisdom. Then, as they’re replying, try to quickly quickly turn their hair black, and you’ll get a better sense of how ridiculously difficult a task is in front of you.