I. “But Why?”
Taiichi Ohno, a Japanese engineer who helped to create the Toyota Production System, once described a five-step system to identify the root cause of a problem. It’s called the Five Whys, and it works like this:
A car doesn’t start. A mechanic begins their diagnosis by asking “Why does the car not start?” and finds the culprit: a dead battery. A good mechanic continues the interrogation and probes deeper, asking, “Why is the battery dead?” It’s because the alternator is broken. The mechanic evaluates each issue as it comes up, one-by-one, and asks “why?” each time. Eventually, several whys down, it becomes clear that the alternator was not maintained on a proper service schedule. This is the root cause.
The trickiest problems that we face in life are often multifactorial, yet we tend to address only the most proximate causes. But when it comes to solving complex problems, diagnosing and addressing them from the onset is usually more effective than firefighting after a spark has grown into an inferno. This is especially true in medicine, where the temporal gap between the initiation of the disease (a random genetic mutation, say) and the appearance of symptoms makes finding the root cause difficult.
This is where a technique like the Five Whys can be applied to great effect.
A recent study in Nature shows the utility of Toyota’s approach. For the study, the authors, led by scientists from Vanderbilt University in Tennessee, investigated type 2 diabetes, a disease most often treated based on proximate causes, such as high blood sugar, and tried to find the root cause. In doing so, they not only illuminated the molecular pathways that lead to type 2 diabetes, but also provided a template for other scientists to follow.
Subscribe to receive essays from Asimov Press.Subscribe
II. The Investigation
Obesity gets most of the blame for diabetes. A high waist circumference, eating too much sugar, and sleep deprivation are all risk factors. According to this view, obesity and these other factors eventually lead, somehow, to pancreatic malfunctioning. Insulin prompts cells to take up sugar from the blood, but in people with diabetes, the pancreas stops making insulin, and the rest of the body begins to resist the insulin signal. A low amount of insulin, combined with insulin resistance, causes high blood sugar. That, in turn, makes the blood toxic and messes up the kidneys, brain, and much else besides.
But there’s a lot missing from this story. Not everyone who is obese has type 2 diabetes, nor does everyone who eats a lot of sugar or carries certain genetic mutations. There’s no smoking gun; merely a lot of circumstantial “risk factors” that sometimes combine to make things go wrong. It is also unclear how these risk factors can cause both the pancreas to stop making insulin and cause cells to resist the insulin.
Unfortunately, less-than-definitive paths of causation are par for the course in medicine. It is difficult to study complex diseases because science is often limited to tightly-controlled environments – and animal models – that don’t always accurately reflect the real world.
For the recent Nature paper, the scientists dug down through the causes of diabetes. For the first of their whys, they asked: Why do beta cells inside the pancreas secrete less insulin?
For decades, scientists had noticed that people with diabetes make less insulin. As the condition progresses, the beta cells that make the insulin proteins dwindle and die off, diminishing insulin levels further. But it’s difficult to understand why.
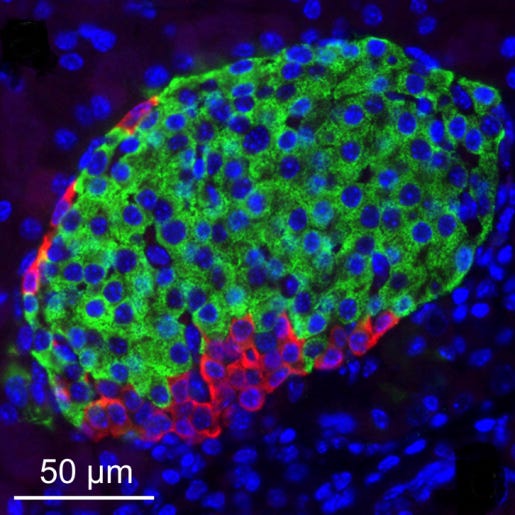
The researchers began by taking beta cells from people with early-stage diabetes and from people without diabetes. Insulin secretion was impaired in the cells from diabetics, but the production and storage of the molecule was not. In other words, the beta cells make a normal amount of insulin, but the molecule is trapped inside. To ensure that this problem stemmed from beta cells themselves and not the pancreas as a whole, the researchers transplanted the diabetic beta cells and the healthy beta cells into healthy mice, and – voila! – the diabetic beta cells still secreted less insulin.
But why? Well, the pancreas is a three-dimensional organ. Cells are not flat; they exist as part of a sprawling network of other cells that surround and support one another. The researchers wanted to see if something might be wrong with this network, so they stained beta cells in the mice and then peered at them using a microscope. They noticed a single difference between the diabetic and non-diabetic pancreas: the former had smaller blood capillaries, which makes it more difficult for nutrients to reach the beta cells.
This finding was confirmed statistically, using something called a “cellular neighborhood study.” In diabetic cells, capillary cells were less likely to be connected to beta cells and more likely to be connected to immune cells, compared to the non-diabetic cells. A lack of nutrients had physically altered the cellular network within the pancreas.
But why? A capillary shrinks over time – it’s a temporal process – but science is often limited to static snapshots. Mice are raised in cages and then sacrificed so that their organs can be studied under a microscope. But this approach merely studies the cells as they exist at one moment in time.
To understand why capillaries shrink in the pancreas, the scientists had to figure out what might have happened before they shrank. So the researchers opted to study the transcriptome, or the record of genes expressed in the diabetic and non-diabetic pancreas. Ideally, the scientists hoped to find some obvious connection, or at least correlation, between genes and outcomes. But they didn’t. The only difference was that early-stage diabetics had slight changes in genes associated with cilia, a hair-like structure that cells use to move water around. Early-stage diabetics also had more cilia in their pancreas.
But why? Again using transcriptomics, the scientists began to look for a “hub gene” that could connect cilia to shrunken capillaries. And they found it in RFX6, a gene that had already been linked to diabetes in other studies. RFX6 is reduced in people with type 2 diabetes and has also been linked to insulin secretion.
One can often “see what a gene does” by knocking it out, or at least diminishing its presence, from a cell. The scientists used a short strand of RNA to “knock down” expression of RFX6 in insulin-producing cells grown in a culture dish. Knocking down the gene did not harm the beta cells, but it did block them from secreting insulin.
Connecting RFX6 to the cilia and capillaries, though, was more challenging. The scientists knocked-down RFX6 in cells yet again, and compared them to cells with normal levels of RFX6. But this time, they did an experiment called single-nucleus multiome profiling. This enabled them to look at every gene that is altered between the two bunches of cells. Cells with knocked-down RFX6 levels had many genes associated with actin filament-based movement and synaptic signaling go up and genes linked to membrane trafficking, cell recycling, and ciliary pathways go down.
In sum: RFX6 is a gene that helps cells dispose of waste. As capillaries shrink, they are less able to remove waste. Cilia levels then increase in an attempt to compensate. People with type 2 diabetes likely secrete less insulin because cells in the pancreas progressively lose their ability to remove waste products, which further damages capillaries and causes them to shrink.
III. Scientific Method
This paper is an important investigation into diabetes. But I would argue that it’s even more important for its methodology.
So many studies, over the long arc of biological research, have stopped at the first “why?” This study, on the other hand, took a full ten years of work and many failed experiments to complete. It’s painful-going to pursue the Five Whys in science, but if we truly want to understand the cause of complex, chronic diseases, such as Alzheimer’s or Parkinson’s, then we almost certainly need to follow such a process.
Medicine is stuck right now because research into all sorts of chronic diseases has been myopic. It is often easiest to target the obvious, proximate cause of a disease, and then focus mechanical experiments on that. Academics striving to keep a regular publishing schedule often trigger, say, Parkinson’s-like symptoms in mice directly through chemical injections, spend a few months treating the mice, and then wrap up the data analysis within 6 months so that a paper can be published by year’s end.
We can do better. Of course, the job of a researcher is often more difficult than that of a mechanic. There are no service manuals for scientific discovery, and scientists are often beholden to unsympathetic funders. Still, we must try to find root causes in biology and medicine, rather than continue to observe epicycles, where sometimes obesity causes diabetes but sometimes doesn’t, and the only way to predict which one it will be is whether Mercury is in retrograde. Messy correlations are a sign of an immature science, and that’s as true today as it was in the early days of modern medicine.
It took more than two-thousand years, from Thucydides’ observations of contagion to Koch’s postulates of microbial infection, for a thorough, root cause analysis of why close contact with an infected person can cause a healthy person to become sick. But hopefully now, with more papers like this one, we won’t have to wait quite so long.